The HL-LHC
The High-Luminosity Large Hadron Collider (HL-LHC) project aims to crank up the performance of the LHC, to increase the potential for physics discoveries after 2029. The objective is to increase the integrated luminosity by a factor of 10 beyond the LHC’s design value.
Luminosity is an important indicator of the performance of an accelerator: it is proportional to the number of collisions that occur in a given amount of time. The higher the luminosity, the more data the experiments can gather to allow them to observe rare processes.
The High-Luminosity LHC will allow physicists to study known mechanisms, such as the Higgs boson, in greater detail and to observe rare new phenomena that might be revealed. For example, the High-Luminosity LHC will produce at least 15 million Higgs bosons per year, compared to around three million from the LHC in 2017.
The HL-LHC upgrade depends on several technological innovations, some of which have already been installed during CERN’s Long Shutdown 2 (LS2).
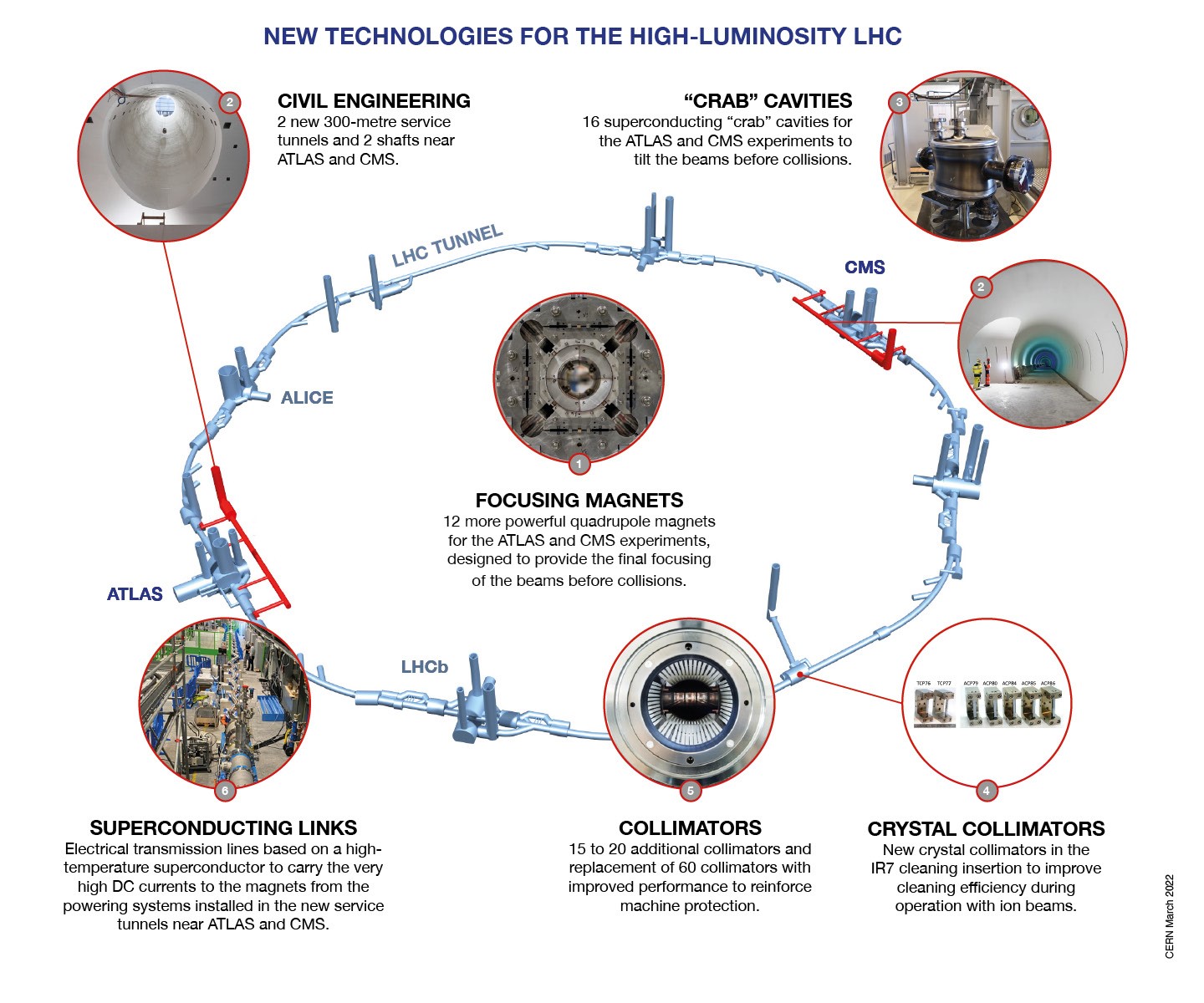
HL-LHC technological innovations
1. Focusing magnets
For the HL-LHC upgrade, more than one kilometre of the LHC machine needs to be replaced. There will be about 100 magnets of 11 new types: four types of main magnets (dipole and quadrupole magnets, which bend and focus the beams), and seven different types of correcting magnets.
In particular, new quadrupole magnets, for the final focusing of the beams before collisions, will be installed in the insertion regions on either side of the ATLAS and CMS detectors. These magnets exploit a key innovative technology providing magnetic fields of up to 12 Tesla. They are built from a novel superconducting material called niobium-tin (Nb3Sn), using a unique design that allows the peak magnetic field strength to be increased by around 50% compared with the current LHC magnets.
2. Civil engineering
The HL-LHC civil-engineering work has centred around LHC Points 1 (ATLAS) and 5 (CMS). Here, most of the equipment required to increase the luminosity for these two experiments will be installed.
At each of the two sites, underground facilities consist of a shaft around 80 metres deep, a service hall that will house cryogenic and other equipment, a 300-metre-long tunnel for electrical equipment such as power converters and four 50-metre service tunnels that connect the new structures to the existing accelerator tunnel.
These four service tunnels will house specific systems to connect the accelerator components to the infrastructures and services in the new HL-LHC galleries, such as radiofrequency transmission lines, superconducting links and cryogenic lines.
Five buildings representing a total surface area of 2800 m2 have been constructed above ground to house the necessary technical infrastructure such as cooling, ventilation, cryogenics and electrical equipment.
3. Crab cavities
Never used before at a hadron collider, a total of 16 compact crab cavities will be installed on either side of ATLAS and CMS during Long Shutdown 3, which is expected to start in 2026 and last for three years.
The superconducting radio-frequency (RF) “crab” cavities enlarge the overlap area of the incoming proton bunches and thus increase the number of collisions. Crab cavities precisely control the tilt of the bunches at the collision point, helping the experiments to fully exploit the scientific potential of the HL-LHC.
4. Crystal collimators
Collimators are designed to absorb all the particles that stray from the ideal beam trajectory, preventing unruly particles from causing damage to sensitive areas of the accelerator.
At LHC Point 7, a first set of Target Collimator Primary Crystal (TCPC) has now been installed. Developed for the HL-LHC project, this jewel of the collimation system offers an alternative way to clean the beam, using silicium bent crystals to deflect the halo particles onto a downstream absorber.
This is a promising technology for the high-luminosity era of the LHC. Previously, crystal collimators have been installed in the Super Proton Synchrotron (SPS) and in the LHC, where they have been tested during the machine development periods, and proved to be very effective, in particular for operation with heavy-ion beams.
5. Collimators
As the HL-LHC will increase the number of collisions, it will also increase the number of potential stray particles, and the existing collimation system must be reinforced.
Collimators close their jaws around the beam to intercept and absorb particles that may stray from the ideal beam trajectory and interfere with the operation of sensitive components. The materials used for the collimator jaws are capable of withstanding extreme pressure and temperatures as well as high levels of radiation.
During CERN’s LS2, 16 new collimators of various types have been installed in the LHC: four primary collimators, eight secondary collimators, and two fixed-aperture passive absorbers. They are all equipped with new sensors that monitor the beam position to allow the position of the jaws to be adjusted quickly and accurately. The primary and secondary collimators feature a new design based on a molybdenum–graphite compound that will help improve the stability of the higher-intensity beams of HL-LHC.
6. Superconducting links
As the future HL-LHC will need magnets with magnetic fields and currents never reached before, superconducting links will be used as part of the electrical transmission system to power these magnets.
A state-of-the-art cabling machine has been developed at CERN to produce components using high-temperature superconductor (HTS) material (REBCO) for connecting these superconducting links to the room temperature power converters. The links themselves use cables of a novel high-temperature superconducting material called MgB2. The term “high temperature” is relative, these superconducting links will allow to transfer currents at temperatures of up to 25 Kelvin (or -239°C) while the nominal operating temperature of the LHC magnets is currently 1.9 K (or -272°C).
High-temperature superconductors have the potential to revolutionise accelerator technology as well as other fields, such as medical applications, magnetically levitated trains, space travel and fusion energy.